last authored: May 2013, Andrew Xiao, Eric Plitman
Overview
The heart is a muscle critical to the function of the cardiovascular system. Its primary function is to pump blood into blood vessels, through rhythmic contractions.
The heart is composed of four major chambers: left atrium, left ventricle, right atrium and right ventricle. The upper two chambers (the left and right atria) receive blood from the body and the lower two chambers (left and right ventricles) distributes blood to the body.
The atria communicate with the ventricles through the ‘atrioventricular (AV) valves’. These valves act like gates and monitor the flow of blood from the atria down to the ventricles. The two atrial cavities are separated from each other by a muscular septum known as the ‘interatrial septum’ and the two ventricular cavities are separated from each other by a thick muscular ‘interventricular septum’.
The right atrium receives deoxygenated blood from the entire body through two large veins, the ‘superior’ & ‘inferior’ vena cavae (SVC & IVC), while the left atrium receives oxygen-rich blood from the two lungs via four ‘pulmonary veins’.
The right ventricle pumps deoxygenated blood towards lungs through a large artery ‘pulmonary trunk’, while the left ventricle pumps oxygenated blood towards the rest of the body through a giant artery known as ‘aorta’. The point from where these large arteries originate from each ventricle is guarded by a valve known as the semilunar (SL) valve. These valves just like the AV valves monitor the flow of blood from the ventricles into the large arteries.
Nodes and valves within the heart sustain the flow of blood within the heart along a designated path, that renders oxygenated blood from the lungs and ultimately, delivers it towards systemic circulation.
Main Vessels and Chambers

Human Heart, courtesy of Yaddah
The heart is composed of four chambers: the right atrium, the right ventricle, the left atrium and the left ventricle. Blood flow through the heart follows a direct path, and the heart’s respective vessels and chambers are responsible for routing this direct flow.
Deoxygenated blood returning from systemic circulation enters into the heart via the inferior and superior vena cavae, which return blood from below and above the heart, respectively. Both of the vena cavae drain into the posterior right atrium. The posterior aspect of the right atrium is composed of a smooth area, in comparison to the muscular anterior region.
Next, blood moves into the right ventricle, as it passes through the tricuspid valve. Here, blood is pumped into pulmonary circulation via the pulmonary arteries. To achieve this movement into the pulmonary arteries, the pressure generated by the right ventricle must exceed that of the pulmonary valve. Via the pulmonary arteries, deoxygenated blood is taken to the lungs, where it is oxygenated.
The pulmonary veins bring newly oxygenated blood back to the heart, depositing into the left atrium. From the left atrium, blood is pumped past the bicuspid valve (also known as mitral valve) into the left ventricle.
The left ventricle is able to generate very high pressures that force the blood past the aortic valve, into the aorta and through the systemic circulation.
Heart Valves
The heart contains four valves that open and close in response to pressure changes that occur as atrial and ventricular chambers of the heart contract and relax. By opening and closing at appropriate times, valves are responsible for maintaining a one-way flow of blood through the heart.

Heart valves, courtesy of OpenStax College
The bicuspid and tricuspid valves, together termed the atrioventricular (AV) valves, are located between the atrium and the ventricle. During ventricular contraction, ventricular pressure causes the closure of the AV valves. Ventricular contraction is also marked by contractions of the papillary muscle, which tighten the chordae tendineae. This prevents the opening of the AV valves and resultant regurgitation of blood into the atria. During ventricular relaxation, the papillary muscles are relaxed, which releases tension in the chordae tendineae and results in the opening of the AV valves. This allows blood to travel along a pressure gradient, moving from the high pressure in the atria to the lower pressure in the ventricles.
The aortic and pulmonary valves are together termed the semilunar (SL) valves. The aortic valve exists between the left ventricle and the aorta, and the pulmonary valve is located between the right ventricle and the pulmonary artery. The SL valves allow the movement of blood into their respective arteries, but prevent backflow into the ventricles. Upon ventricular contraction, pressure increase in the ventricles. When the pressure in the ventricles exceeds the pressure in the arteries, the SL valves open, and blood enters the pulmonary and systemic circulation. When the ventricles relax, and the pressure inside of them decreases, blood flows back to the heart. In response, the SL valves shut and block further entry of blood into the heart.
Cellular Structure
The heart consists of three layers: a layer of epicardial and connective tissue; the myocardium, a thick layer of contractile cardiomyocytes, and the thin endocardium, composed of endothelial cells. Myocytes comprise only 25% of the number of heart cells but account for almost 90% of its volume. The remainder are mostly endothelial cells of the capillary network and fibroblasts. Extracellular matrix and leukocytes are rare.
The heart consists of three layers. The outermost layer made up of connective tissue is known as epicardium, a middle thick layer made up of specialized cardiac muscle fibers known as, myocardium, and a thin membranous innermost layer made up of flat endothelial cells, known as endocardium.
The major bulk of the heart is composed of the contractile cardiomyocytes (myocytes). This type of muscle is only found in the heart and nowhere else in the body, hence the name ‘cardiac muscle’. Cardiac muscle is similar to skeletal muscle in many ways. Both types appear striated as a result of the arrangement of actin & myosin filaments (the filamentous proteins responsible for contraction).
Cardiomyocytes measure up to 25 um in diameter and 100 um in length. They are arranged in a circumferential and spiral orientation around the left ventricle. Each cell contains numerous myofibrils, which are long chains of sarcomeres. Sarcomeres are the fundamental contractile unit of the cell, being made up of actin and myosin.
Within each cardiomyocyte, sarcomeres line up, producing the characteristic cross-striated banding pattern. The cell membrane is termed to sarcolemma. Deep invaginations of the sarcolemma called T tubules project into the cell, increasing contact with the extracellular environment and facilitating rapid conduction of calcium influx and contractility. Gap junctions between cells are termed intercalated disks and stain darkly on light microscopy. Most calcium is stored with the sarcoplasmic reticulum.
Given their tremendous energy demands, cardiomyocyte volume is approximately 35% mitochondria, located between individual myofibrils.
The high metabolic activity of cardiomyocytes is supported by a rich blood supply. This is described further here.
Pericardium
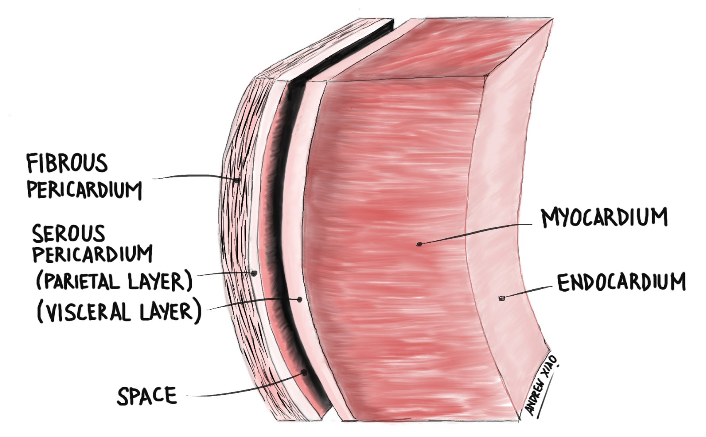
courtesy of Andrew Xiao, author
The heart is contained in the pericardium, a tough, double-layered membranous sac. The innermost layer, called the visceral pericardium (or epicardium), is in direct contact with the heart muscle proper (myocardium). External to the visceral pericardium are the parietal pericardium and fibrous pericardium (they are fused together, with the fibrous layer being the most external layer of the pericardium). Between the visceral and parietal pericardium is a slit-like potential space, called the pericardial space (or pericardial cavity). This space is filled with pericardial fluid, which acts as a shock absorber as well as a lubricant to allow the heart to beat smoothly.
It is important to note that the visceral and parietal pericardium are actually continuous with one another, forming a continuous membrane called the serous pericardium. To provide a visual analogy for the way the serous pericardium wraps around the heart, imagine the serous pericardium as a water-filled balloon and the heart as a closed fist. Now slowly push the fist against the balloon until the balloon has wrapped around the fist. The area of balloon directly in contact with the fist is equivalent to the visceral layer of the serous pericardium, while the area of balloon not in contact with the fist is the parietal pericardium.