Authors: Paige McParlan, Jennifer Tomasone
Introduction
image courtesy of Patrick Lynch
The cardiovascular system is comprised of the heart, blood vessels (or vasculature) and blood. It functions to pumps blood around the body, providing a steady supply of oxygen, energy, and nutrients while simultaneously removing wastes.
The heart is at the core of the cardiovascular system, and is responsible for pumping blood along the almost 100,000 km of blood vessels. The pumping action of the heart is under electrical control, where specialized nerve bundles and fibres deep in the heart tissues work together to cause the different parts of the heart to contract in sequence. In order for blood to circulate, there must be a degree pressure in the cardiovascular system. Blood pressure is the force within the vasculature that allows blood to flow from areas of high to low pressure.
Heart Overview
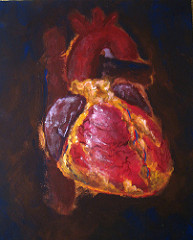
courtesy of Denn
The heart is considered to be the “workhorse” of the body. It is a muscular, fist-sized organ weighing 250-350 grams. It is a remarkably efficient and reliable pump, propelling over 6000 litres of blood daily, and beating approximately 35 million times yearly, or over 2.5 billion times in a 70 year lifetime. It is continuously contracting, and only rests during the millisecond pauses between heart beats.
The heart lies centrally within the thoracic cavity, approximately between the 2nd and 6th ribs. The top of the heart can be found at the same level as the sternal angle on the sternum in the body. The bottom of the heart, also known as the apex, sits at the xiphisternal angle, located on the xiphoid process.
The heart sits in the pericardial sac, within the pericardial cavity – the space between the two lungs. This sac is filled with fluid, which reduces the friction that occurs when the heart beats. The pericardial sac also helps to anchor the heart in its position.
Heart Structure
main article: heart structure
The heart is a four-chambered organ, with each chamber having a one-way valve that opens as blood moves out of the chamber. The two top chambers, the atria, receive blood from either the vena cavae (right atrium) or from the lungs (left atrium). The atria are responsible for moving blood into the lower chambers, the ventricles, on their respective side. The ventricles eject blood either to the lungs (right ventricle) and the aorta (left ventricle). The general term for the one-way valves that separate the atria and the ventricles is atrio-ventricular (AV) valves. The general term for the one-way valves that separate the ventricles from the lungs or body is semilunar valves.
Deoxygenated blood from the upper and lower body enters the right atrium through the superior and inferior vena cavae, respectively. Once the right atrium contracts, the deoxygenated blood passes through the tricuspid valve to enter the right ventricle. From there, blood is pumped through the pulmonary valve to the pulmonary arteries that lead into the lungs, where blood is oxygenated.
Oxygenated blood from the lungs travels through the pulmonary veins to return to the left atrium. Once the left atrium contracts, the oxygenated blood passes through the mitral valve into the muscular left ventricle. In order to reach the body’s tissues, the left ventricle contracts, causing the aortic valve to open, allowing the blood to enter into the aorta. The aorta is responsible for distributing oxygenated blood to all of the body’s tissues.
The heart as a pump
main article: heart as a pump
The atria and ventricles work together to distribute deoxygenated blood to the lungs (via the pulmonary circulation) and oxygenated blood to the body (via the systemic circulation). In order to do this, coordinated contractions of the atria and ventricles alternate,.
The right and left atria contract at the same time. During atrial contraction, the AV valves open and the ventricles are relaxed allowing them to fill with blood from the atria. Atrial contraction ends when the AV valves (the tricupsid valve on the right and the mitral valve on the left) close, createing S1 – the “lub” sound that is associated with the “lub-dub” heartbeat. Closure of the AV valves ensures that blood does not regurgitate into the atria when the ventricles contract.
Like the atria, the right and left ventricles also contract at the same time. During ventricular contraction, the semilunar valves open so that blood can be pumped to either the pulmonary arteries (through the pulmonary valve on the right) or to the aorta (through the aortic valve on the left). Ventricular contraction ends when the semilunar valves close due to back pressure, creating S2 – the “dub” sound that we associate with our “lub-dub” heartbeat. Closure of the semilunar valves ensures that blood does not re-enter the ventricles once it enters pulmonary and systemic circulation. Once ventricular contraction is completed, atrial contraction begins once again.
The cardiac cycle is the period of time from the end of one heartbeat through the end of the next heartbeat. In other words, one cardiac cycle occurs in the time between the very beginning of atrial contraction (just after the semilunar valves close) to the very end of ventricular contraction (including the closure of the semilunar valves).
Stroke volume is the total volume of blood which is pumped by the heart in one cardiac cycle. It is measured in milliliters (mL) per heart beat. Cardiac output is the volume of blood pumped by the heart during a given period of time. Cardiac output can be calculated by multiplying heart rate (beats per minute) by stroke volume (mL/beat). At rest, the heart pumps all the blood in body through it in only one minute (cardiac output is close to 5 L per minute). For example, if the heart pumps ~70 mL of blood in one cardiac cycle (e.g., stroke volume = 70 mL), and one’s heart rate is 70 beats per minute, one’s overall cardiac output would be 4900 mL/minute.
Electrical Control of the Heart
main article: electrical control of the heart
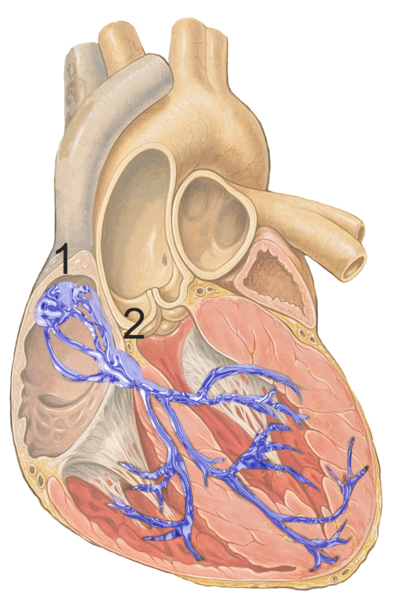
1: sinoatrial node; 2: atrioventricular node used with permission, J Heuser
Embedded within the heart is an electrical conducting system, which facilitates the coordinated contraction of the atria and ventricles, and ensures continuous cardiac cycles.
Electrical communication in the heart begins with an action potential generated by autorhythmic cells, which spontaneously and rhythmically depolarize to fire action potentials. This action potential begins in the sinoatrial (SA) node located in the superior portion of the right atrial wall. The action potential wave then travels through an electrical conducting system comprised of non-contractile autorhythmic fibers or nerve cells. The SA node is connected to the atrioventricular (AV) node (located at the centre of the heart between the two atria and two ventricles) by a branched internodal pathway. From the AV node, Purkinje fibers (specialized conducting cells) transmit the electrical signals down the atrioventricular (AV) bundle (also called the bundle of His), found in the ventricular septum (the muscular wall between the right and left ventricles). At the apex of the heart, the AV fibers divide into right and left bundle branches, which extend over the right and left ventricles, respectively.
After the depolarization wave occurs in the electrical conducting system, it is directly followed by a wave of contraction in the heart’s muscular tissue. The electrical signal is transmitted between adjacent cardiac muscle fibres via gap junctions in intercalated disks. These intercalated disks allow for fast and coordinated contraction of all of the heart’s muscle cells surrounding the electrical conducting system. When the SA node sets off an action potential, the signal is sent to all muscle fibres in the right and left atria, causing atrial contraction. When the electrical signal reaches the AV node, the atrial muscle fibres begin to repolarize and relax. As the AV node continues to transmit the action potential through the Purkinje fibres and into the right and left bundle branches, the electrical signal is sent to all muscle fibres in the right and left ventricles, causing ventricular contraction. Once ventricular contraction occurs, the ventricular muscle fibres relax. The next cardiac cycle begins when the SA node fires another action potential.
The SA node cells set the pace of the heartbeat, and are thus referred to as pacemaker cells. However, there are other cells such as the Purkinje fibers, that under certain conditions can also act as pacemakers. Since their rhythm is significantly slower than the pace of the SA node, they do not usually set the heartbeat. If the SA node becomes damaged and can no longer set the pace, one of the slower pacemakers then takes over.
Electrocardiograms (ECGs or EKGs) show the summed electrical activity which is generated from all of the cells of the heart. There are three major waves which can be seen on a normal ECG. They are the P wave, QRS complex and the T wave. The P wave is the first wave, and corresponds to atrial depolarization (or contraction). The next two waves, together called the QRS complex, represents ventricular depolarization (or contraction) as well as atrial repolarization. The final wave, the T wave, represents the repolarization of the ventricles. ECGs can be analysed to help understand irregularities in the electrical conduction of the heart.
Blood Pressure
main article: blood pressure
Blood pressure is the force exerted by blood on the walls of the blood vessels. This pressure is created by contraction of the heart’s ventricles, which is the driving force for blood flow throughout the body. When blood is ejected from the left ventricle into the aorta, the aorta and arteries expand to accommodate it. When the ventricle closes, the elastic arterial walls recoil, causing blood to be propelled forward into smaller arteries and arterioles.
Blood pressure is usually measured in the systemic arteries. During ventricular contraction, the pressure in the aorta reaches an average high of 120 mmHg (systolic pressure). During ventricular relaxation, blood pressure in the aorta falls to an average low of 80 mmHg (diastolic pressure). The stored energy in the elastic wall of the aorta permits the diastolic pressure to remain greater than 0 mmHg. The rapid increase in pressure when the ventricle contracts is felt as a pulse.
Blood pressure is highest in the aorta and decreases continuously as blood flows through the vasculature due to friction between the blood cells and the blood vessel walls. Once blood has reached the veins, it is at very low pressure, and a pulse can no longer be felt. This low-pressure blood must then return to the heart. Some veins have internal, one-way valves that ensure that blood cannot flow backwards once it has passed the valve. Venous return is also aided by the contraction of skeletal muscle, which compresses veins and forces blood past the valves and closer to the heart.
Blood pressure is a function of cardiac output x total peripheral resistance (BP = CO x TPR). Blood pressure is therefore increased with:
- An increase in blood volume
- An increase in salt or electrolytes circulating in the blood (which causes an increase in blood volume due to osmosis)
- Constriction of the arteries and arterioles, which causes more resistance to blood flow, so the left ventricle must work harder and eject blood with more pressure to push the blood through the vessels
- Dilation of the veins and venules, which causes more blood to remain in the vessels so less blood is being pumped out of the heart.
Blood pressure control is mediated by a complex series of factors, including the sympathetic nervous system, circulating hormonal factors, and local metabolites. These are all described under the main topic of blood pressure.
Vasculature
The branching network of blood vessels carries blood from the heart to the tissues of the body. Placed end-to-end, the body’s blood vessels would stretch almost 100,000 km.
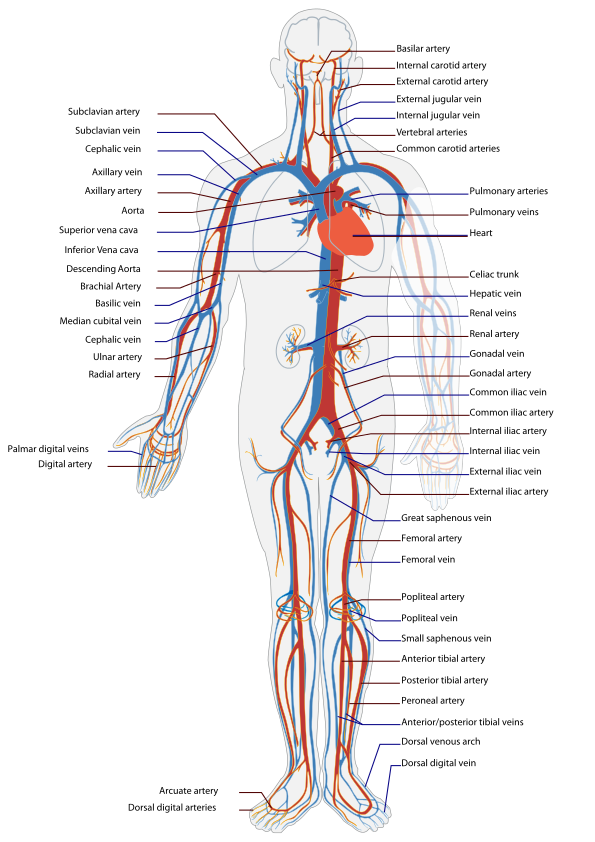
Human vasculature, courtesy of Mariana Villarreal
Arteries (and arterioles) transport blood from the heart to capillaries, where nutrient and waste diffusion occurs. Veins (and venules) carry blood back to the heart via a system of valves.
The enormous branching of the vasculature creates large differences in cross-sectional area and flow. Arteries are the thick-walled vessels transporting blood away from the heart to tissue beds. Their elastic walls allow them to stretch under pressure and provide constant blood flow to capillaries. The aorta, which branches off the heart’s left ventricle, is the largest artery and is roughly 2.5 cm in diameter.
As blood travels from the aorta to arteries to arterioles, both the diameter of the vessels and vessel wall thickness (and thus elastic properties) decrease. Average arteries have a diameter of 4.0 mm and a wall thickness of 1.0 mm, while arterioles have an average diameter of 0.3 mm and a wall thickness of 0.06 mm.
Capillaries are the smallest vessels, made up simple, porous endothelial cells. It is here that gases, nutrients, and wastes diffuse between the cardiovascular system and the tissues. To allow for this exchange, capillaries have a large surface area, and its walls are only composed of a single layer of cells. The average diameter of capillaries is 0.08 mm.
From the capillaries back to the heart, blood passes through first through venules, and then through veins. The diameter and wall thickness of these vessels both increase as blood gets closer to the heart. Average venules have a diameter of 0.2 mm and a wall thickness of 0.01 mm, whereas average veins have a diameter of 5.0 mm and a wall thickness of 0.5 mm.
Veins contain most of the systemic circulation blood volume due to their large compliance, giving them the name ‘capacitance vessels’. This is not due so much to the elastic properties of their walls, but rather their ability to change shape.
Blood Flow Around the Body
Blood is able to circulate through the body because of pressure gradients, where fluids flow from regions of higher pressure to regions of lower pressure. The heart creates high pressure when it contracts, which allows blood to flow out of the heart (where pressure is highest) to the closed loop of blood vessels and capillaries (where the pressure is the lowest). This allows for the continuous circulation of blood that we see in the cardiovascular system.
Most (85%) of the body’s 5L of blood is in the systemic circulation, with 10% in the pulmonary circulation and 5% in the heart. The systemic veins contain about 65% of the body’s blood.
Hemodynamics describes blood flow throughout the vessels of the cardiovascular system. Blood vessels that have larger diameters (i.e., the aorta) will exert less friction on blood flow from their vessel walls, resulting in a high blood velocity through these vessels. However, vessels with smaller diameters exert more friction on blood flow. The smaller the vessel diameter, the more friction the blood passing through will experience, and the slower the blood flow will be. This slowing of blood flow is useful in the capillaries where slower blood flow means more efficient exchange of oxygen, carbon dioxide, nutrients, and wastes. However, in order to compensate for the volume of blood that must be transported through blood vessels at any given time, the number of capillaries is large so that the overall cross-sectional area of capillaries can accommodate the passage of 5000 mL of blood every minute. This means that total blood flow through each type of vessel (arteries, arterioles, capillaries, venules, veins) must be 5000 mL/minute (or equal to the individual’s cardiac output).
The distribution of blood in the systemic circulation varies according to the metabolic needs of the tissues. For example, skeletal muscle at rest requires less blood flow than skeletal muscle during exercise. Likewise, the digestive system requires more blood flow to digest food after a meal. In general, the number and size of arteries leading to an organ is associated with the amount of blood it requires for normal functioning. Below is a listing of average blood flow per minute to each of the body’s major organs and tissues at rest.
Cardiac Output | 5000 mL/min |
Brain | 70 mL/min |
Heart muscle | 20 mL/min |
Liver and digestive system | 1350 mL/min |
Kidneys | 1000 mL/min |
Skeletal muscle | 1050 mL/min |
Skin | 25 mL/min |
Bone and other tissues | 45 mL/min |
Development of the cardiovascular system begins to develop during the third week of gestation when the embryo can no longer satisfy it’s nutritional requirements from diffusion alone. All components of the cardiovascular system (including the heart, vasculature, and blood) are derived from the embryo’s mesodermal germ layer. Of note, the cardiovascular system during prenatal life relies on placental circulation (where the fetus receives oxygenated blood from the placenta), and thus structural changes of the heart and vessels occur after birth to accommodate the cessation of placental blood flow and the beginning of respiration.
Resources and References
University of Utah WebPath images of the heart.
Canadian Cardiovascular Society
Silverthorn, D. U. (2012). Human Physiology: An Integrated Approach (6th Edition). Toronto: Pearson. ISBN-10: 0321750071